Advancing structural performance of aerospace heat exchangers
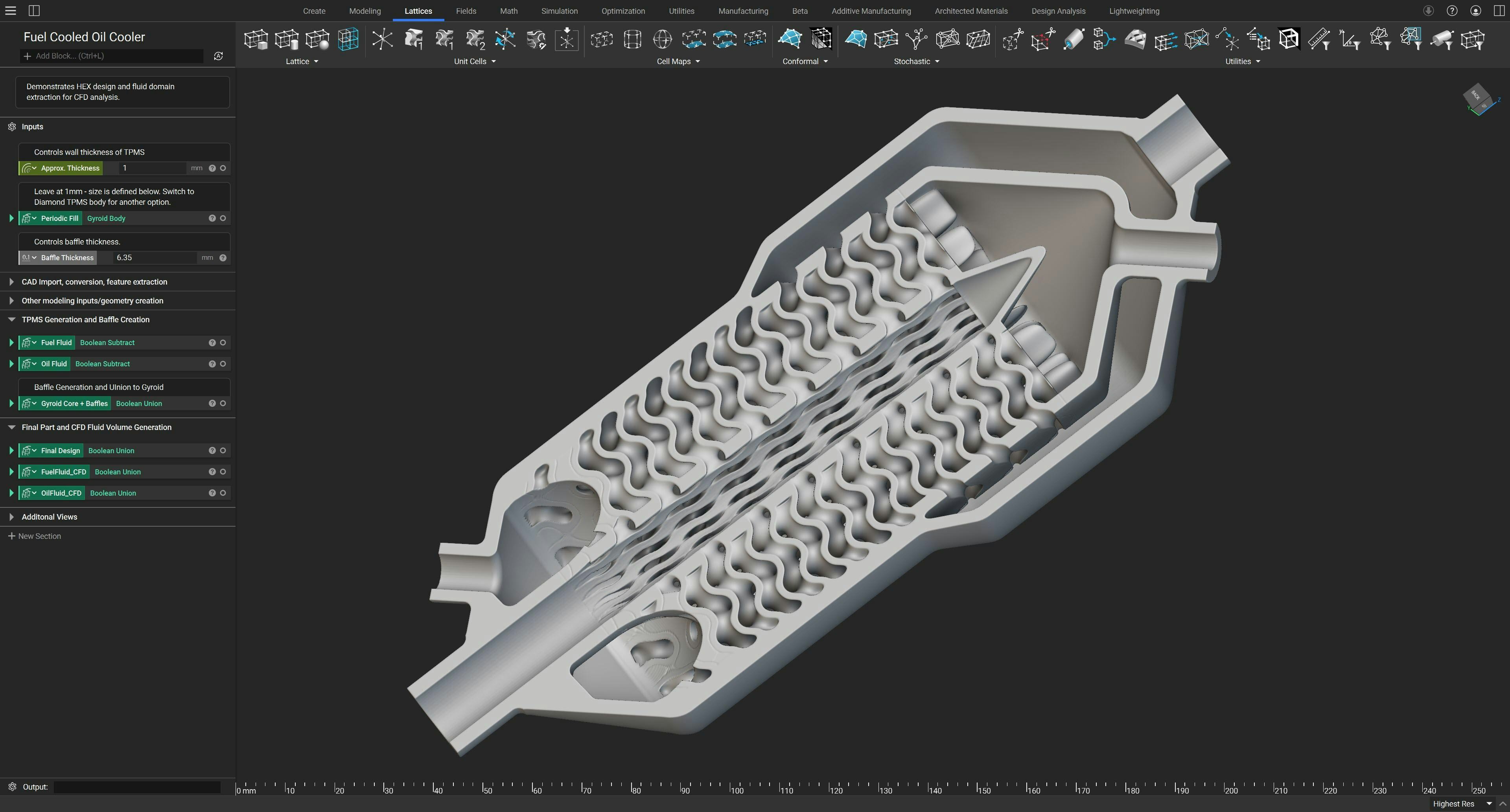
Written by Alejandro Carcel Lopez | Staff Application Engineer at nTop
Published on October 30, 2023
Applications
- General
- Thermal management
Key Software Capabilities
- Design automation
- Lattice structures
- Simulation
Dive into an exploration of how additive manufacturing (AM) can enhance the structural performance of aerospace heat exchangers. Learn how nTop, in tandem with AM, turns design challenges into opportunities and influences the future of aerospace solutions.
In aerospace, the structural integrity of heat exchangers directly impacts their efficiency.
Additive manufacturing can help enhance this structural performance. By enabling techniques like structural optimization and part consolidation, and in conjunction with advanced design software like nTop, engineers can produce heat exchangers that are both robust and efficient. This combination of design and technology paves the way for heat exchangers that set new benchmarks in performance. In this article, we will delve deeper into the intricate relationship between structural design, additive manufacturing techniques, and the potential they bring to the aerospace industry.
Current challenges for aerospace heat exchangers
Rising technological demands pose challenges for heat exchangers. Engineers are constantly devising ways to enhance structural performance, efficiency, and resilience, ensuring heat exchangers can adapt to dynamic aerospace conditions while optimizing thermal management and minimizing potential failure points. Let’s explore some of the key challenges:
Design intricacies
The need for compactness due to space constraints in aerospace systems often conflicts with the design features required for optimal thermal performance. Crafting a design that balances these elements is a challenge. Additive manufacturing has great potential to deliver geometries that find a better balance, but exploring and achieving those geometries with traditional CAD tools is very difficult. However, platforms like nTop offer advanced capabilities that allow engineers to refine and integrate advanced 3D-printable structures seamlessly.
Traditional heat exchanger designs, such as the shell and tube model, can introduce further complications. Multiple components increase the probability of component failure and maintenance burden, such as o-ring seal failure.
Material selection and understanding
Aerospace heat exchangers are subjected to extreme conditions, from high altitudes to the scorching heat of engine compartments. Choosing materials that can withstand these conditions while maintaining their structural and thermal properties is crucial.
To conduct accurate simulations, engineers must understand the properties of the materials they’re testing. This depth of knowledge enables precise predictions regarding a component's ability to endure specific stress loads.
Burst duct events
These sudden, unpredictable spikes in fluid pressure can lead to catastrophic failures if not accounted for in the design. It's not enough for heat exchangers to be built for average or predictable operational stresses — they need to be resilient enough to withstand harsh conditions like burst duct events.
Rapid technological advancements
With technology constantly evolving, heat exchangers need to be designed with adaptability in mind, ready for upgrades or integration with newer systems as they emerge. For instance, as propulsion technologies evolve, the requirements for thermal management can shift, demanding heat exchangers that can handle different temperature ranges or fluid types. Structural performance also becomes paramount as these shifts can introduce new stress factors and operational conditions. Additionally, new generation heat exchangers may also be required to act as structural components as well as a heat transfer system, which makes structural integrity all the more relevant.
How additive manufacturing can advance structural performance of heat exchangers
AM gives engineers the flexibility to conceptualize and create designs that were too complex for traditional manufacturing methods.
AM is particularly beneficial when designing parts for unique aerospace conditions. Engineers can design intricate internal geometries with precision, resulting in heat exchangers that excel both in function and form. Advanced structures manufacturable by AM such as TPMS gyroid and diamond lattices, thanks to their quasi-isotropic and high-surface area characteristics, are great at both bearing loads and heat transfer, making them ideal candidates to develop novel heat exchangers that excel at load and heat transfer.
nTop enables the design of these intricate geometries. It seamlessly integrates simulation tools, promoting an iterative design approach to optimize performance in real-world conditions.
Benefits of additive manufacturing in structural performance
Part consolidation and reduced assembly complexity
Complex designs often lead to a greater chance of component failure. Traditional heat exchangers, with their many tubes, fasteners, and seals, can be prone to problems like oil or fuel leaks. These issues increase safety risks and maintenance needs. Using additive manufacturing to consolidate parts can address these challenges. AM boosts structural reliability by minimizing separate parts.
Additionally, by consolidating multiple components into simpler integrated designs, engineers can design efficient heat exchangers that are both smaller and lighter. This not only lowers the risk of component malfunctions but also simplifies the assembly process.
Customization and flexibility
In an industry where one-size-fits-all rarely fits all, additive manufacturing allows engineers to create tailored solutions, fine-tuning heat exchangers to meet specific criteria and optimize performance. From altering the size and shape to optimizing fluid flow, AM provides greater flexibility. Through AM, engineers can improve structural performance while adhering to stringent aerospace requirements.
Complex geometries
Traditional manufacturing methods are often constrained by their inability to produce intricate designs. By enabling the fabrication of complex geometries that are crucial for enhanced heat transfer efficiency, AM takes thermal management and structural performance to new heights.
These internal structures can dramatically improve the strength-to-weight ratios of aerospace components, which is crucial for the overall structural integrity and thermal performance of heat exchangers.
Material efficiency
Traditional manufacturing methods, such as subtractive processes, often begin with a bulk of material and remove excess to shape the desired part, leading to significant material wastage. This approach also incurs higher costs and environmental impacts.
AM builds parts layer-by-layer, adding material only where necessary, substantially reducing waste. Moreover, the capability to produce intricate internal geometries without molds or tooling means even complex designs can be fabricated without excess material.
Rapid prototyping
Unlike traditional methods, which often require time-consuming processes like tooling, AM allows engineers to move from a design concept to a tangible prototype quickly. This immediate turnaround not only enhances the efficiency of the design process, but also enables iterative improvements in a fraction of the time traditionally required.
Leverage your additive manufacturing capabilities with nTop
As the aerospace industry continues to demand more efficient and high-performing components, engineers need tools to help them meet these rigorous standards. nTop elevates additive manufacturing capabilities, enabling engineers to design components with precision and performance.
Ribbing and latticing
One way nTop can help improve the structural integrity of components is through ribs and surface lattices. Introducing ribs or surface lattices can effectively increase the surface area of a component. In the context of heat exchangers, an increased surface area provides added rigidity and resistance to bending or deformation. By using ribbing, engineers can reduce the thickness of the main body of a component without compromising its strength. The ribs provide the necessary structural support, ensuring the component is both lightweight and robust.
Design automation
nTop's design automation features streamline the design process, ensuring consistency and efficiency. By encoding expertise into a block-based visual programming environment, engineers can scale and optimize their design processes. This, coupled with scripting capabilities, facilitates rapid iterations on lattice characterization. As a result, the design and analysis process is expedited, enabling engineers to swiftly land on a design that aligns with both performance and safety benchmarks.
Advanced modeling
Sharp corners or interfaces in a design are notorious areas of stress concentration that can lead to premature failure. nTop has unique design features, such as booleans with automatic blend radius, to blend geometries and eradicate these sharp interfaces. This ensures a smoother stress distribution throughout the component.
Beyond just improving structural integrity, nTop's advanced modeling capabilities grant engineers a higher degree of design freedom. They can experiment with complex geometries, merge multiple components into cohesive units, or introduce innovative features without the constraints of conventional design tools such as parametric errors, disconnected surfaces, a need for manual repair, long geometry re-generation times or software crashes.
Learn more about aerospace and defense heat exchangers in our latest guide, or find out how nTop can improve your approach to engineering design and manufacturing by reaching out to us today.
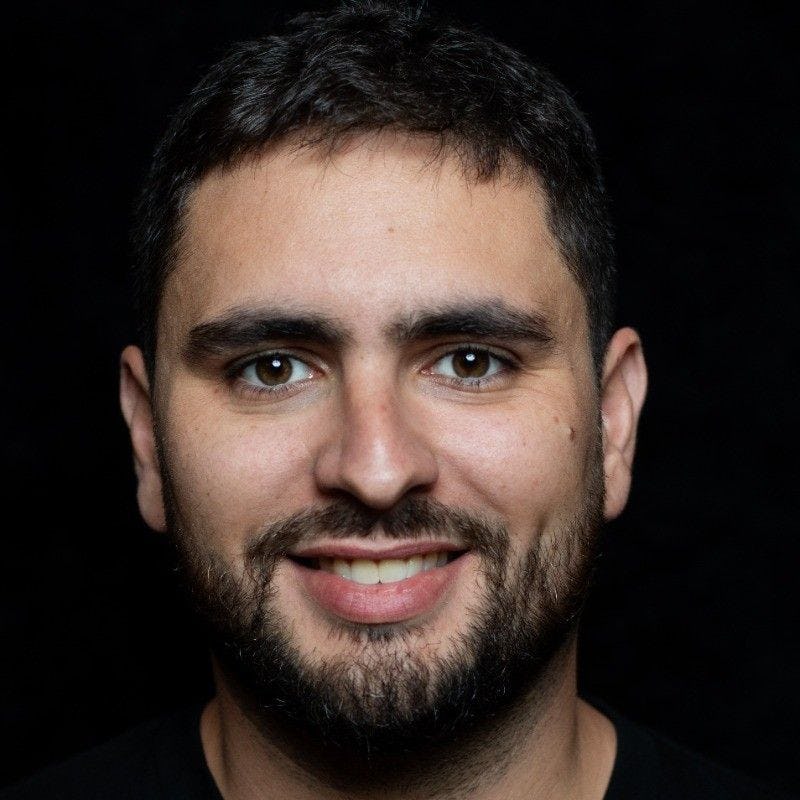
Alejandro Carcel Lopez
Staff Application Engineer at nTop
Alejandro is an MSc. Aerospace Engineer working in additive manufacturing and innovation for lightweighting and thermal management across several industries, mainly aerospace. He is experienced in the certification and qualification of aircraft propulsion systems and structures integration, design operational reliability, stress, thermal, and flow studies.
Related content
- VIDEO
Creating a computational design workflow to lightweight drone panels with nTop’s new ribbing tools
- WEBINAR
Accelerating Product Engineering with Computational Design
- VIDEO
nTop Siemens Energy at CDFAM NYC 2024
- CASE STUDY
Reducing weight to help win and withstand the rigors of a 24 hour Le Mans race
- ARTICLE
An nTop intern’s experience: Learning computational design and creating a lightweight drone